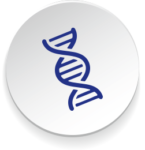
Post Electrophoretic Analysis Articles
Radioactive Emissions and the Use of Isotopes in Research
Radioactive decay occurs with the emission of particles or electromagnetic radiation from an atom due to a change within its nucleus. Forms of radioactive emission include alpha particles (α), beta particles (β), and gamma rays (γ). α particles are the least energetic, most massive of these decay products. An α particle contains two protons and two neutrons, and thus comprises a stable helium nucleus. α particles only weakly penetrate whatever matter they encounter. They are unable to penetrate even 10 cm of air.
Beta particles are high energy electrons. These are produced during the conversion of a neutron to a proton in the nucleus. β particles are emitted in concert with a neutrino (neutrinos are almost impossible to detect). The sum of the energies of the neutrino and β particle is a constant for a given isotope, and defines the maximum energy (Emax) which can be observed for any particle emitted from that isotope. Emax is approached only for particles emitted with a low energy neutrino. In practice a distribution of energies is observed, which is characteristic for the emitting isotope.
Gamma-rays differ from α and β emissions in that γ-rays are electromagnetic radiation, not particles. γ -rays are quite penetrating, in many cases passing through up to 5 cm of lead. Additionally, γ -rays are capable of generating secondary β emission from material they pass through. An electron in the material may absorb the energy of the γ-ray, and be promoted to an excited state which is no longer bound to its nucleus. Such an electron escapes from the atom as a free β-particle.
Characteristics of Useful Isotopes
The list of known radioisotopes is extensive, but the number of isotopes used in research is fairly small. To be useful as a label in research, an isotope must meet a restrictive set of qualifications. First of all, it must be an element that is already a part of the experimental system. For biological research, for example, isotopes of carbon, hydrogen, oxygen, and phosphorus are widely used. Alternatively, an element which can be substituted for another in the system may be used: for example, sulfur isotopes can be used in place of oxygen.
Useful isotopes must also have a reasonable half-life. The half-life of an isotope is a measure of the rate at which it decays to a nonradioactive state. As each nucleus emits its radiation, it eventually reaches a stable configuration which will not emit again. Thus a given quantity of isotope will eventually yield a finite total amount of radiation.
Each nucleus decays independently, so the probability of a decay event occurring at any time is equal to the probability of any one nucleus decaying multiplied by the total number of radioactive nuclei present. This number changes as decay progresses, always proportional to the number of radioactive nuclei remaining. To express a rate of radioactive decay which is independent of the amount of material involved, the time required for the decay of 50% of the starting material, the half-life, is a useful quantity. Half-lives may range from milliseconds to thousands of years, a value characteristic of the particular isotope. Assuming that a 24 hour period is required for an experiment, an isotope with a half life (t1/2) of 6 hours would undergo 24 fold reduction in emissions, or a loss of 94%. Most experimentally used isotopes have half-life values of 10 days or more.
To determine the number of radioactive atoms present within a sample at a given time, use the equations below.
λ = 0.693 / t1/2N = N0e-λt
t1/2 = half-life
N = number of radioactive atoms
N0 = initial number
t = elapsed time
Isotope | Emission | Energy (MeV) | Half-Life (t1/2) |
---|---|---|---|
Tritium (3H) | β | 0.019 | 12.3 yrs. |
Carbon (14C) | β | 0.156 | 5730 yrs. |
Sulfur (35S) | β | 0.167 | 87.2 days |
Phosphorus (32P) | β | 1.710 | 14.3 days |
Phosphorus (33P) | β | 0.249 | 25.3 days |
Iodine (125I) | γ | 0.178 | 59.9 days |
Using Isotopes in Research
For many kinds of research, the utility of radioisotopes stems from their chemical identity with their nonradioactive counterparts. This allows their incorporation into "tracers," radiolabeled components which can be followed and detected through a series of reaction steps. Tracers are invaluable in metabolic studies, where they allow the determination of the catabolic and/or anabolic fates of nutrient compounds. Animals are fed diets containing labeled molecules, such as sugars or amino acids, and the radioactivity is followed through the system until excretion or incorporation. Another use for isotopes has been protein and DNA analysis studies, where probes which bind to specific macromolecules can be radiolabeled without interfering with their activity.
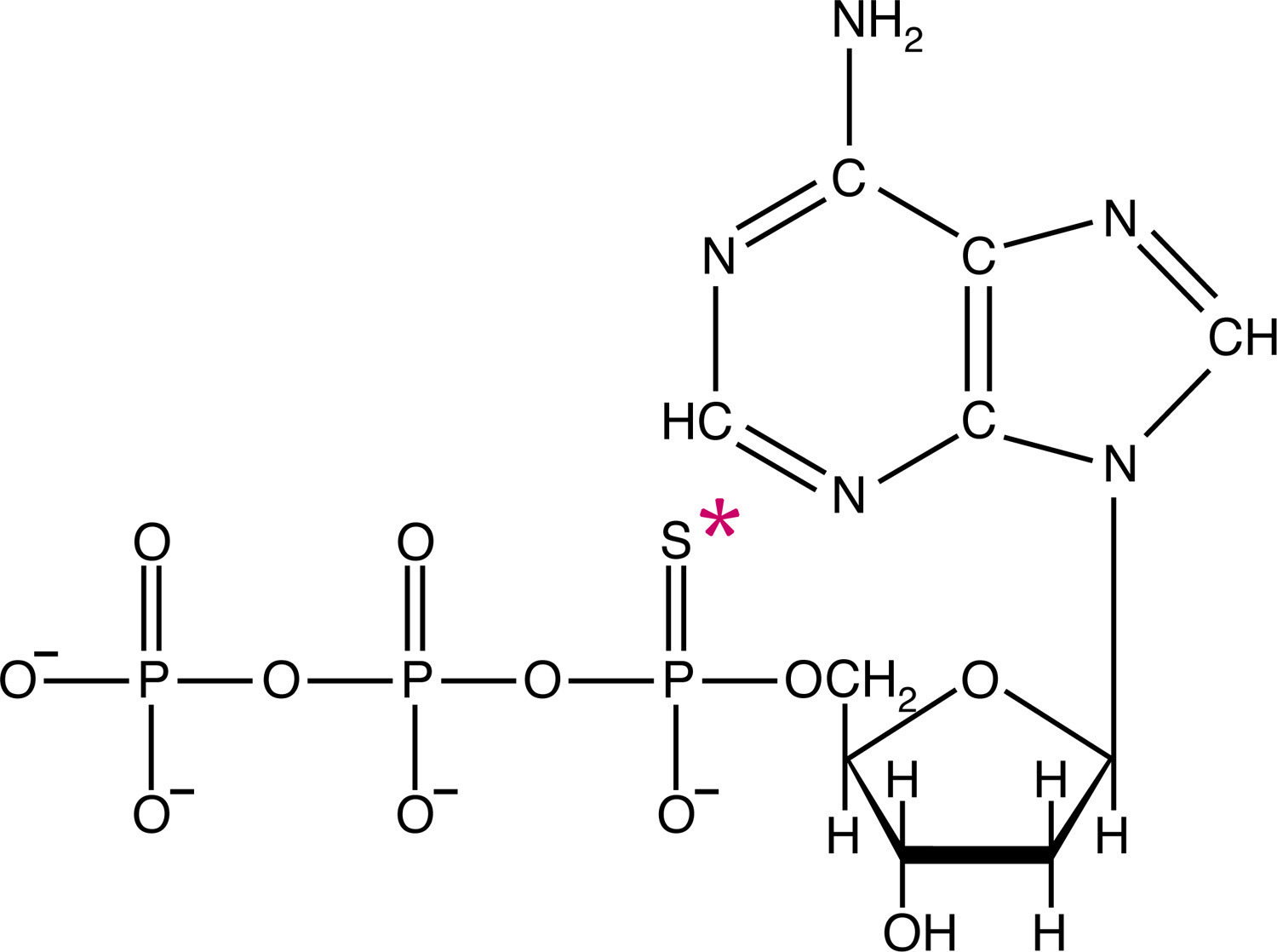
Examples of radiolabeled biological molecules including tritiated glycine and ATP labeled with either 35S or 32P.
NEXT TOPIC: Measurement of Radiation and Isotope Quantitation
- The Polyacrylamide Matrix-Buffer Strength
- The Polyacrylamide Matrix
- The Mechanical and Electrical Dynamics of Gel Electrophoresis — Electrophoresis System Dynamics
- The Mechanical and Electrical Dynamics of Gel Electrophoresis – Ohm’s Law
- The Mechanical and Electrical Dynamics of Gel Electrophoresis – Intro and Sample Mobility
- The Electrophoresis Matrix
- The Agarose Matrix
- Radioactive Emissions and the Use of Isotopes in Research
- Multiphasic Buffer Systems
- Horizontal and Vertical Gel Systems – Vertical Tube Gels
- Horizontal and Vertical Gel Systems – The Vertical Slab Gel System
- Horizontal and Vertical Gel Systems – The Horizontal Gel System
- Homogeneous Buffer Systems
- Faint bands, low background
- Faint Bands, High Background
- Ethidium Bromide Staining
- Electrophoresis Buffers-Choosing the Right Buffer
- Electrophoresis Buffers–The Henderson-Hasselbalch Equation
- Coomassie Blue Stain- Troubleshooting
- Buffer Additives-Surfactants
- Buffer Additives-Reducing Agents
- Buffer Additives-Hydrogen Bonding Agents
- Biological Macromolecules: Nucleic Acids
- Biological Macromolecules – Proteins